Although the problem of missing mass was noticed seventy-five years ago (Zwicky 1933, Oort 1940), the nature of this mass is not yet known. Non-baryonic dark matter represents about 23% of the content of the universe. It is made of unknown particles, which do not emit or absorb light, but are detected indirectly through their gravity. Dark matter in nearby galaxies has a flat radial profile in the center, that is called a core. This is in contradiction with simulations made in the standard model of cold dark matter (CDM), which predict steep radial profile, or cusps. A solution to this problem has been proposed with warm dark matter (WDM) or self-interacting dark matter, that can create cores. However, WDM predicts smaller cores for higher mass systems, in conflict with observations.
Since the beginning, constraints have been put on the possible mass of any dark particle : due to the decrease of the coarse-grained phase space density or PSD for non-interacting particles, their number density is bounded, implying that their mass must be larger than 1 Mev, according to Tremaine & Gunn (1979). This is to account for the maximum phase-space density observed today in dwarf spheroidal galaxies. Since then many authors have revised this constraint, and the minimum mass is a fraction of keV (e.g. Dalcanton & Hogan 2001, Madsen 2001, Boyanovsky et al 2008, Boyarsky et al 2009).
The PSD of the particles decrease from an initial value at the time of decoupling. The temperature of the universe at decoupling Td is still a free parameter. Particles can decouple as ultra-relativistic, if Td mc2, or as non-relativistic if Td << mc2. In both cases, the mean distance they can run from decoupling is called free streaming length. CDM candidates feature a small free streaming length favoring a bottom-up hierarchical approach to structure formation, smaller structures form first and mergers lead to clustering on the larger scales. When the free streaming length is non-zero at the time of structure formation (z 3300) it imposes a minimum mass scale for structures, where smaller masses cannot form. This is the case for the WDM.
The present work considers that the DM could be warm, with a free streaming that allows formation of galaxies (see Figure 1). With some assumptions on the history of the DM PSD in the non-linear regime, giving some constraints on the way the PSD has decreased, it is possible to derive bounds on m and Td. They estimate that the decrease in the PSD is between 1 and 10 000 for dwarf spheroidal galaxies dSphs. More accurate analysis of N-body simulations should narrow this range which depends on the type and size of the galaxy considered. The mass of the dark matter particle, is in the keV scale and the temperature when the dark matter particles decoupled is in the 100 GeV scale at least. Dark matter particles could be fermions or bosons (cf Figure 1).
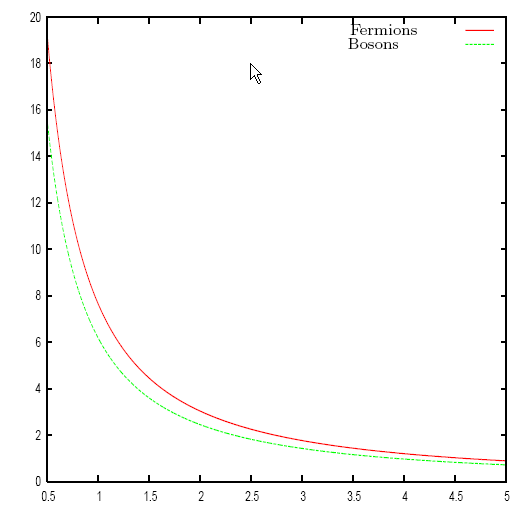
In the same framework, lower and upper bounds for the dark matter annihilation cross-section are derived. Given the constraints from the observations (X-rays, optical or lensing observations), the dark matter non-gravitational self-interaction is negligible.
Reference H. J. de Vega, N. G. Sanchez, `Model-independent analysis of dark matter points to a particle mass at the keV scale’, 2010, MNRAS, in press
Other literature Dalcanton J.J., Hogan C.J. : 2001, ApJ 561, 35 Boyanovsky, D., de Vega, H.J., Sanchez, N.G. : 2008, Phys. Rev. D77, 043518 Boyarsky A., Ruchayskiy, O., Iakubovskyi, D. : 2009 JCAP 03, 005 Madsen J. : 2001, PhRvD, 64b7301 Oort, J. : 1940 ApJ, 91, 273 Tremaine S., Gunn J.E. : 1979 PhRvL 42, 407 Zwicky, F. : 1933 Helv. Phys. Acta, 6, 124
Contact
Norma Sanchez (Observatoire de Paris, LERMA, et CNRS)