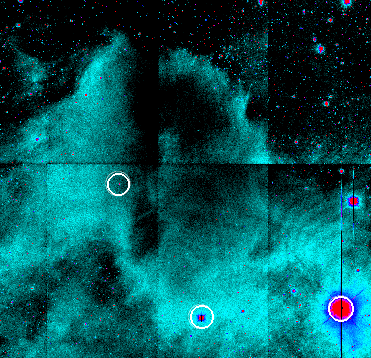
Figure 1 : I band image of L183 cloud taken at CFHT, Canada-France-Hawaii Telescope. Note in the center a dark zone, obscured by dust (absence of background stars), surrounded by scattered light, represented by the blue nebulosity. Three stars in the field are circled in white, to serve as beacons on the other images. Click on the image to enlarge it Stars form inside interstellar clouds of gas and dust and small clouds like L183 form low mass stars, like our Sun. How do they do that ? This is the main question. To understand star formation, one needs to observe inside these clouds, which, full of dust, are totally opaque to visible light. The clouds’ content is revealed to us by the emission or absorption of dust at different infrared wavelengths, as shown in this Spitzer image (Fig. 2) taken at 8 µm. It reveals the absorption of the light emitted by « PAHs » (PolyAromatic Hydrocarbons, small particles of « dust » or large molecules made of cyclic carbon chains) at the cloud’s surface : the light emitted on the backside of the cloud reaches us after a strong attenuation due to the dust inside which thus reveals itself (Figure 2).

However, the study of dust is difficult and moreover, it gives no information on the gas motions in the cloud, especially those which could reveal a starting collapse leading to the formation of a star or the rotation of the cloud, prelude to the creation of protostellar disks, the sites of planet formation. Only high velocity resolution spectroscopic study of the gas can provide us with such information. One has to find which gas component can serve this purpose. The main component, hydrogen, present in its molecular form in dark clouds, is not directly observable and neither is helium. Since the end of the 60s, the existence of other molecules has been attested, among which carbon monoxyde (CO) and water (H2O) are the most abundant. CO has revealed itself to be a good gas tracer but in the mid 90s, as foreseen 20 years before, it was shown that this species would disappear in dark clouds under certain conditions : when the cloud is cold (< 20 K) and thick enough to be protected from hard UV radiation (coming form all the stars around). Then, most molecular species deplete onto dust grains to form ice mantles. Water is probably the first one to stick to grains (water freezes at an anomalously high temperature with respect to its molecular weight) and molecules such as CO, CS, SO turn into ice layers onto grains as soon as the extinction in the cloud reaches 10 magnitudes (in the visible). There is then no way to study the heavily buried cores with these tracers. Surrogate species have been searched for, which would better resist to these extreme conditions. Though we don’t yet understand why, molecules bearing only nitrogen and hydrogen atoms seem to be able to remain in such very dense cores. Among these species, one is well-known on Earth, namely ammonia (NH3), and another one, ionized, is more specific to the interstellar medium (N2H+).

Figure 3 : Contours of the N2H+ emission in L183 are superposed on the image of Figure 2. The emission is strongly correlated with the maximum absorption zone of the cloud. Click on the image to enlarge it With the help of a radiative transfer model (model which tries to compute the signal emitted by the molecules to compare it to what has been observed), it has been possible to deduce the parameters which best describe the prestellar core at the center of this image (a second core, clearly visible within the N2H+ contours is situated just north of it. It is behind in terms of evolution towards forming a new star). The core is colder than what was considered as a cold core up to now, being no more than 7 K over absolute zero. In this extreme coldness, and far inside the cloud, even the N-bearing molecules disappear from the gas phase, which means that either they or their mother molecules (like N2) deplete onto grains. In this extremely cold region, where turbulence disappears too, all conditions seem to be realised to have a collapse starting, leading eventually to the formation of a new star. NH3, N2H+, and N2D+ spectra which have allowed this analysis are shown below (Figs. 4, 5 and 6).



Réference Depletion and low gas temperature in the L183 prestellar core : the N2H+ - N2D+ tool Laurent Pagani (LERMA, Obs-Paris), Aurore Bacmann (OASU, Bordeaux), Sylvie Cabrit (LERMA, Obs-Paris), Charlotte Vastel (CESR, Toulouse) Astronomy & Astrophysics, in press
Contact
- Laurent Pagani
(Observatoire de Paris, LERMA et CNRS ) - Sylvie Cabrit
(Observatoire de Paris, LERMA et CNRS )